Article of the
Month - December 2020
|
A Toolset for Automating 1mm Measurement
Accuracy in Photogrammetry Surveys
This article in .pdf-format
(11 pages)
Ken Varner, Wolfgang Herbst, And David Schmidhuber, Germany
SUMMARY
The implementation of calibrated photogrammetry
tags produced with optimal surface materials enables very precise
engineering surveys (down to 1mm relative measurement accuracy) of water
management infrastructure. Such photogrammetry tags create
automatically detected control points within the model space that also
provide the basis for very precise measurement control.
Each unique tag is coded within the
photogrammetry software completely automating the typical manual work of
adding control points, merging projects, and merging different datasets
of the same project. The nuance presented here is using the coded tags
around the surveyed object as the measurement system. Producing the
tags with an optimized surface material enables the surveyor to mimic
measurement standard conditions (lab conditions) in the field to
systematically achieve sub-cm measurement accuracy.
The recipe for success for implementing this
coded tag based measurement system as well as case studies of 3D surveys
with 1mm measurement accuracy of stone infrastructure using Unmanned
Aerial Vehicles (UAVs) and handheld cameras are presented here. The key
elements to take into account for successful implementation of such
surveys are the following:
-
Know your camera
-
Trust your measurement
-
Control your space
-
Understand your photogrammetry software
The correct implementation of photogrammetry
tags with consumer grade measurement devices and consumer grade UAVs and
cameras enables very precise and very fast photogrammetry based 3D
models at a much lower cost compared to laser scanners and total
stations.
1. INTRODUCTION
The advent of consumer grade Unmanned Aerial
Vehicles (UAVs) and multicopters weighing less than 2 kg has created a
surge in new technologies specifically for surveying and inspection. In
particular, photogrammetry has achieved a wide spread market and is now
considered a standard survey and inspection tool within these
industries’ overall toolset. Here, we present a toolset consisting of
consumer grade equipment to systematically automate precision
photogrammertry workflows.
The four main aspects that one must consider in
order to obtain down to 1mm accurate measurement photogrammetry models
are listed here and will be described more in depth within this paper:
-
Know your camera
-
Trust your measurement
-
Control your space
-
Understand how your photogrammetry software
works
The two most commonly reported metrics in a
calibrated photogrammetry surveys are pixel resolution (most commonly
referred to as Ground Sampling Distance (GSD)), which determines the
overall visual accuracy of the model, and the measurement accuracy,
which can be presented in several ways. Even though the GSD has an
effect on determining the baseline for measurement accuracy, GSD and
measurement accuracy are controlled and determined completely
independent of one another. The GSD is determined by the visual
properties relating to the camera, distance from object, and focal
length. Measurement accuracy is determined by the measurement system
used within the model space.
2. KNOW YOUR CAMERA
The main input for photogrammetry based surveys
and inspections are images. In this case, we are presenting results
based on standard RGB imagery from consumer cameras. The camera’s
physical properties as well as the image distance from the model object
are the key factors in calculating the GSD. In Figure 1, the key
parameters for the GSD calculation are defined.
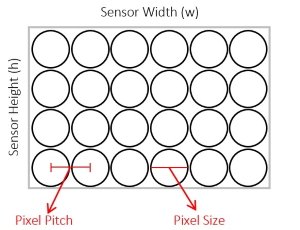
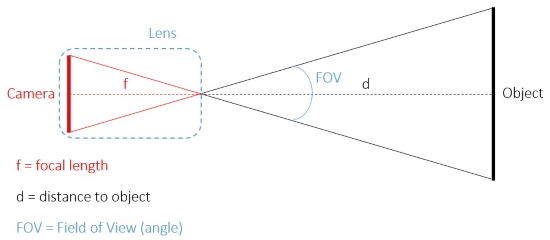
Figure 1: The camera’s physical
properties used for calculating GSD.
With these parameters defined, it is possible to
now calculate a camera system’s GSD with the common formula below:
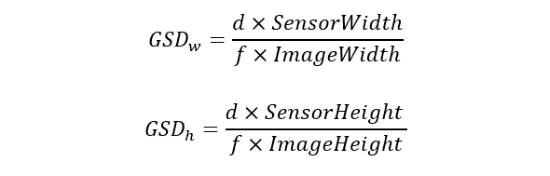
In common practice, one then selects the higher
of the two values for determining the GSD. In this project, a consumer
grade Sony a5000 camera with an APS-C sensor was used with a 10 mm
lens. This camera system’s corresponding properties for photogrammetry
applications are listed here: Using the calculations from above, we can
calculate that a maximum distance of 2.33 m from the model object is
necessary in order to maintain a GSD of 1 mm.
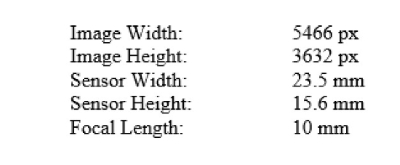
3. TRUST YOUR MEASUREMENT
It can be very challenging for human beings to
verify measurements in the real world, especially as the measurements
get smaller across larger spaces. For this reason, we rely on certified
equipment such as laser distance measurement tools, Total Stations, and
laser scanners to precisely measure the world around us. Even though
such devices are certified for accuracy, it is still the responsibility
of the user to maintain the boundary conditions necessary to provide
best accuracy while applying the proper techniques in the field.
For this work, we used a Leica Disto 810 for
measuring at 1 mm accuracy.
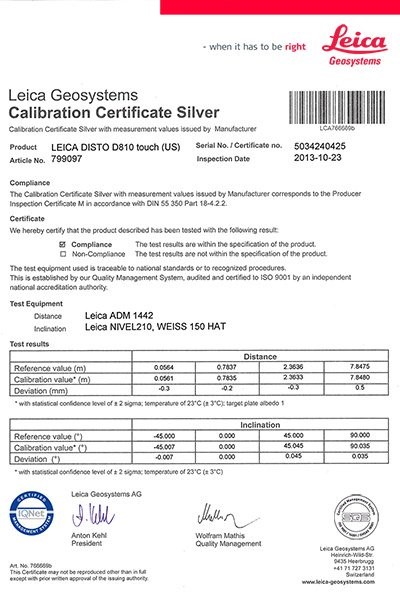
Figure 2: Calibration
certificate for a Leica Disto 810
The Calibration Certificate of Figure 2 shows
that a “target plate albedo 1” was used to calibrate the device. This
now becomes the baseline for providing a measurement system around our
model object. By creating diffuse reflective surfaces for targeting our
DISTO device, we can achieve optimal surface conditions for precise
measurements.
4. AUTOMATED TAGGING
An important practice when performing precision
photogrammetry is the use of coded targets (also known as tags or
markers) that can be automatically registered in the photogrammetry
software. Figure 3 shows an example of Chilitags[1] as they are
automatically detected and registered within the photogrammetry
software. Coded targets are commonly used for automating the
photogrammetry workflow and provide the following benefits:
automatically generate tie points, automatically locate Ground Control
Points, reduce time for manual processing steps, and seamlessly merge
sub-projects.
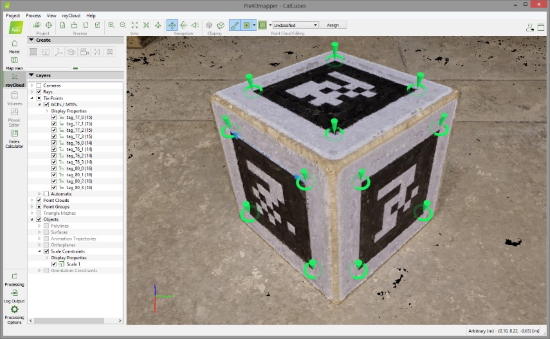
Figure 3: Automatically
detected coded targets within the Pix4D[2] software.
This concept is not new, but the nuance
presented here is using the coded tags around the surveyed object as the
measurement system. Producing the tags with an optimized surface
material enables the surveyor to mimic measurement standard conditions
(lab conditions) in the field to systematically achieve sub-cm
measurement accuracy. The combination of precisely locating the coded
targets’ corners while using an optimized surface material for precise
measurements with the DISTO device enables 1 mm measurement precision
for terrestrial photogrammetry projects.
5. CONTROL YOUR SPACE
The idea of ”controlling your space” is that
much of the infrastructure being modelled with photogrammetry is not
optimal for pulling precise measurements due to surfaces materials,
shapes, or inaccessibility. By implementing coded targets printed on
diffuse reflective surfaces around the infrastructure to be scanned, we
create predictable points for pulling precise measurements independent
of the conditions of the model space. Figure 4 shows an example of 4
cubes being placed around a stone bridge creating a precise measurement
system within the model space. The points used for measurements are
then automatically recognized within the photogrammetry software for
model calibration.
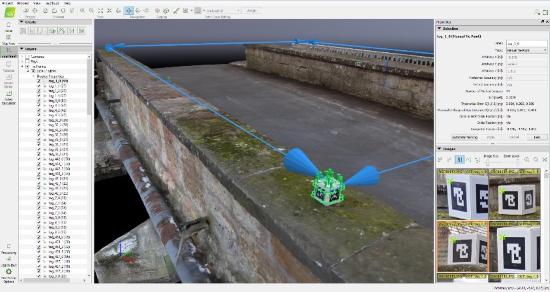
Figure 4: Use of coded targets
on aluminum cubes to create a predictable measurement space around the
model object.
6. FIELD RESULTS
6.1 Stone Wall
A photogrammetry model of the stone wall in
Figure 5 was contracted with the goal of finding the combiner stones
that provide the mechanical stabilisation of such a wall. These stones
are single stones that traverse both sides of the stone preventing wall
collapse over time. In order, to locate these stones, it was necessary
to first, create a 1 mm accurate survey model of the structure and
second, create calibrated, mirrored orthophotos of the two sides for a
precise overlay of both sides of the wall in order to ”see through” the
wall and determine which stones traverse the entire structure.
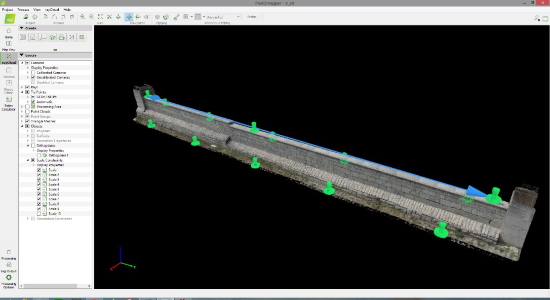
Figure 5: Final mesh of the
stone wall within Pix4D.
Note the control points in green, which were
automatically detected in the software, and the scale constraint across
the top of the wall.
Figures 6 and 7 show the GSD and measurements
results of the photogrammetry model, where a GSD of 1 mm was achieved
and a measurement accuracy sigma of 0.6 mm over 27.160 m.
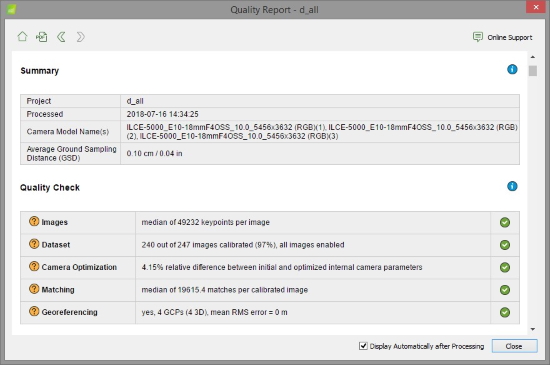
Figure 6: Quality Report of the
photogrammetry model.
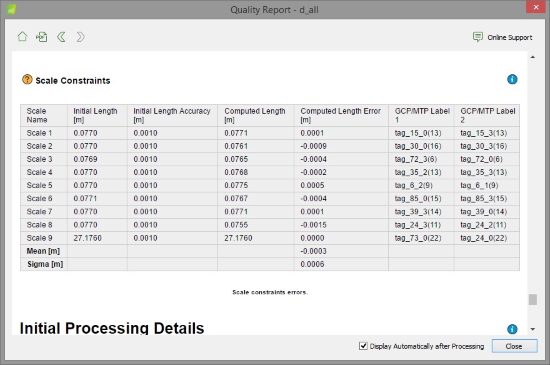
Figure 7: Quality Report of the
photogrammetry model showing the errors of the compiled length
measurements and scale constraints.
6.2 Stone Bridge
A photogrammetry survey of the stone bridge in
Figure 8 as part of a renovation project. The following results were
achieved: GSD = 1.3 mm ; Measurement accuracy = ±1 mm.
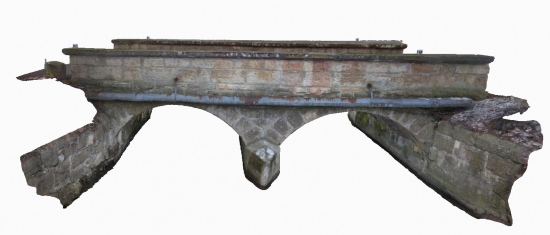
Figure 8: Final mesh of a stone
bridge
6.3 Dam Inspection
A photogrammetry survey of the dam in Figure 9
as part of a renovation project. The following results were achieved:
GSD = 1.7 mm ; Measurement accuracy = ±1.2 mm.
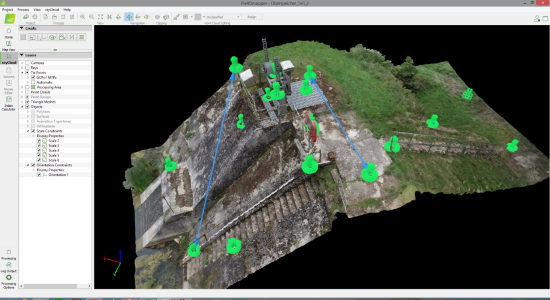
Figure 9: Photogrammetry model
of a dam within Pix4D.
All green control points were automatically
detected using coded targets.
REFERENCES
-
Chilitags: Robust Fiducial Markers for
Augmented Reality. Q. Bonnard, S. Lemaignan, G. Zufferey, A. Mazzei,
S. Cuendet, N. Li, P. Dillenbourg. CHILI, EPFL, Switzerland.
http://chili.epfl.ch/software. 2013.
-
www.pix4d.com
BIOGRAPHICAL NOTES
Dr. Ken Varner has a background in semiconductor
measurement physics and has spent the last several years applying
photogrammetry as a precision measurement tool in the built
environment. With several patents in the aerial inspection space, Ken’s
focus is on automated precision of aerial inspection workflows.
CONTACTS
Dr. Ken Varner Vaireco
GmbH Von-Emmich-Str. 4 Konstanz
GERMANY
Tel. +49 1716983117